At the prestigious New York Learning Hub, Engineer Derek Nnaemeka Aloy-Amadi, a renowned expert in materials science, and polymer engineering presented an essential study that explores the groundbreaking role polymers are playing in modern engineering. His research, Advanced Applications of Polymers in Engineering: Innovations and Future Perspectives, demonstrates how polymer technology is transforming key industries…
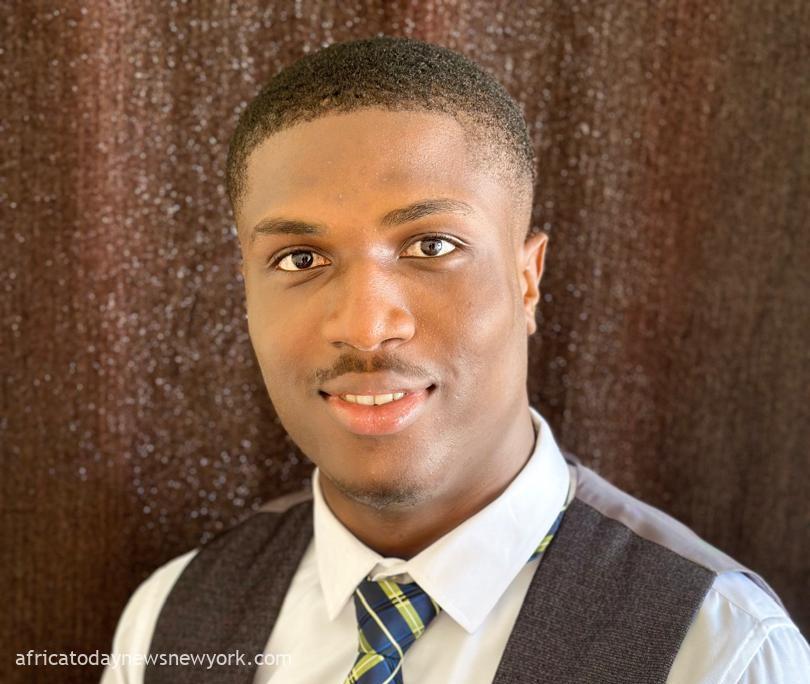